Published on April 12, 2016
Case Introduction
Patient is a 54 year old male who presented at the ER complaining of abdominal pain for the past two days. He admitted to the consumption of a 12 pack of beer four to five nights a week for the past two weeks. He began vomiting during the morning of his admittance, and the vomit turned bloody as he was waiting in the ER. Patient was awake and alert but complained of light headedness and had no chest pain. His stool was dark colored and had no blood in it. Blood pressure was 70/40 and pulse rate was markedly elevated at 120 bpm. Respiration rate and temperature were normal. Abdominal examination revealed an enlarged liver and patient exhibited epigastric pain. A complete metabolic panel, prothrombin time, and partial thromboplastin time tests were ordered upon admittance.
Both prothrombin and partial thromboplastin times were normal, revealing no coagulation abnormalities. Electrolytes and plasma proteins were stable. Blood urea nitrogen was elevated, giving a BUN/creatinine ratio over twice the normal value. Elevated BUN levels indicate gastrointestinal bleeding because large amounts of hemoglobin within red blood cells that have been introduced into the intestines are digested and taken into the bloodstream as amino acids, which contain nitrogen.
The patient is in hypovolemic shock with a significant amount of blood volume lost. Fluid resuscitation is begun immediately with administration of Ringer’s lactated solution. Ringer’s is an isotonic crystalloid solution with lactate added to correct possible metabolic acidosis in the patient. An initial volume of three liters is administered, which will replace approximately 750 ml of blood volume. If the patient’s vital signs do not respond to initial fluid resuscitation, further amounts of intravenous fluid should be administered. Once fluid resuscitation has begun, a sample of the patient’s blood should be taken to be typed. Once blood has been typed, packed red blood cells of the corresponding antigen group should be administered to the patient in order to restore oxygen carrying capacity of the blood.
Intravenous administration of proton pump inhibitors can be started during fluid resuscitation in order to decrease the risk of a re-bleed post-resuscitation. The somatostatin analog octreotide should be administered intravenously to reduce blood flow through the gastrointestinal vasculature and reduce the bleeding rate. Once the patient’s vital signs have stabilized, the bleeding site should be identified and treated. A nasogastric lavage can be used to clear the stomach contents of blood and determine if bleeding is ongoing, but is not a necessary procedure in hypovolemic shock cases. Endoscopy should be performed to identify the site of bleeding, and the endoscope itself can be used to administer one of several different therapies to stop the bleeding. If endoscopic therapy is insufficient to stop bleeding, surgical intervention may be necessary.
Upper Gastrointestinal (UGI) Bleeding
Hemorrhage of the gastrointestinal tract in the portion of the esophagus, stomach, or duodenum is defined as upper gastrointestinal bleeding. UGI patients may present with blood in their vomit as well as melena or hematochezia. Melena is the passage of dark colored stool as a result of excess hemoglobin entering the upper digestive tract, whereas hematochezia is the passage of fresh blood in the stool and is usually only a result of rapid UGI bleeding or lower gastrointestinal bleeding.
UGI bleeding has many causes, and patient history is critical in determining etiology. Peptic ulcers are the most common cause of UGI bleeding, and their development is caused primarily by overuse of non-steroidal anti-inflammatory drugs (NSAIDs) or infection by the bacteria Helicobacter pylori. Ulcers are breaks in the mucosa of the gastrointestinal tract and are often chronic. Hemorrhage of gastrointestinal varices, severe gastric inflammation, and tears in the intestinal lining caused by physical trauma are other common causes of UGI bleeding. Sudden inflammation of the gastric lining, known as acute gastritis, may result in UGI bleeding due to the weakening of the gastric mucosa. Varices are abnormally dilated blood vessels and are especially prone to rupture. Upper gastrointestinal tears are of two types: Mallory-Weiss and Boerhaave tears. Mallory-Weiss syndrome occurs when severe vomiting or coughing fits cause the mucosa of the gastroesophageal junction to tear. Boerhaave syndrome is a tear in the esophageal mucosa superior to the gastroesophageal junction.
Since the patient has received no medical examination in over 20 years, his lifestyle history will be important in determining the cause of his UGI bleeding. If the binge drinking reported in the last two weeks is not regular behavior, then acute gastritis should be suspected. If heavy drinking has been a habitual behavior for the patient, his liver function should be assessed as alcoholic liver disease may be the cause of his bleeding. Liver disease will lead to decreased organ function and portal hypertension. Increased portal venous pressure can lead to backflow of blood into the gastrointestinal vasculature, which can result in the formation of varices. If the liver is dysfunctional, aminotransferase levels should be elevated, albumin levels will be low, and a delayed prothrombin and partial thromboplastin times should be observed.
Alcohol and Gastrointestinal Bleeding
Acute gastritis can be caused by the excessive consumption of alcohol. High alcohol intake can also agitate existing ulcers or varices and lead to hemorrhage that would have otherwise not occurred. Fermented alcoholic beverages, such as beer, directly stimulate acid secretion in the stomach as well as an increase of gastrin levels in the blood, but only consistently in non-alcoholic individuals. [i] Increases in gastrin levels will stimulate acid secretion into the stomach by the parietal cells. The causative agent for this increase in stomach acidity is likely a byproduct of alcoholic fermentation, as fermented drinks lose this effect once they have been distilled, but has yet to be identified. The patients increased consumption of beer over the past two weeks may have induced his gastrointestinal bleeding.
Blood Clotting and Prothrombin Time
Blood clotting, or coagulation, is one of the body’s homeostatic mechanisms for cessation of bleeding in the event of a disruption in the endothelial lining of the blood vessels. Upon damage to an endothelial wall, physiological changes in the endothelial cells as well as exposure of blood to the extracellular matrix triggers the coagulation cascade. There are two main steps in the clotting cascade: platelet plug formation, and formation of a fibrin clot. Plug formation is facilitated by the activation of platelets, small membrane-bound cell fragments that circulate in the blood, whereas fibrin clot formation can be initiated by two mechanisms, either the intrinsic or extrinsic clotting pathway.
Platelets, found in the plasma, are produced by the fragmentation of large cells called megakaryocytes that develop and fragment in the bone marrow. They are anucleate and possess several types of granules containing important coagulation factors. The endothelium acts to keep platelets inactive in undamaged vessels by physically separating them from the extracellular matrix and by secreting prostacyclin, a prostaglandin that acts directly on platelets to inhibit their activation. When a break in the endothelium occurs, platelets are exposed to collagen in the extracellular matrix. Contact with collagen activates platelets, which now degranulate and release serotonin, ADP, thromboxane A2, and von Willebrand factor. Von Willebrand factor strongly cross links glycoproteins on the platelet surface to collagen molecules, so the force of blood flow does not wash them away from the site of injury. Serotonin causes vascular spasm, which constricts blood vessels and reduces blood flow to the damaged site. ADP and thromboxane A2 mediate further aggregation of platelets at the site of plug formation. On activated platelets, the phospholipid phosphatidylserine begins to appear oriented toward the extracellular layer of the plasma membrane more so than the intracellular layer and will act to increase binding of certain coagulation factors discussed later. The platelet plug has now been formed.
There are many factors involved in fibrin clot formation, each with distinct functions in the clotting cascade. Most factors circulate as inactive serine protease precursors. Serine proteases all contain a serine in their active domain, hence the name, and cleave peptide bonds through hydrolysis. These proteases have specificity for their substrates, which are usually other inactive coagulation factors. A few clotting factors serve as cofactors in certain reactions during coagulation. All clotting factors are found in the plasma, and the majority of them are produced by the liver. These are fibrinogen, prothrombin, and factors V, VII, VIII, IX, X, and XI. Liver hepatocytes are responsible for the production of most coagulation factors; however, it is now clear that factor VII is produced by the endothelial cells lining liver sinusoids[ii]
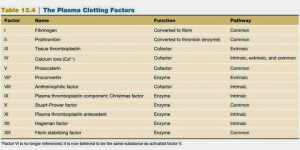
Use this table as a reference during discussion of the clotting pathways (All factors categorized as enzymes here are serine proteases)
The extrinsic and intrinsic clotting pathways both end in a common pathway for fibrin clot formation by activation of factor X, but through different cascades. During the clotting discussion, a lower case “a” will follow the roman numeral of a clotting factor to indicate that it is in the active form e.g. factor X was activated to factor Xa. Use this flowchart as a guide during the description of each pathway.
The extrinsic pathway is triggered by tissue injury and is mediated by tissue factor, a glycoprotein located on the membranes of leukocytes, cells composing the tunica media and externa of blood vessels, and subendothelial tissue cells such as smooth muscle and fibroblasts. Upon injury to the endothelial lining, tissue factor is exposed to the coagulation factors in plasma. Tissue factor acts as a receptor for factor VII, allowing it to be activated. Factor VIIa now associates on an activated platelet membrane along with tissue factor, factor X, and calcium. These assembled components are referred to as the extrinsic tenase complex, named so because it leads to the cleavage of factor X by factor VIIa. The extrinsic tenase complex will form on an activated platelet as opposed to an inactive circulating platelet because of the upregulation of negatively charged phosphatidylserine on the surface. Activation of factor X is where the intrinsic and extrinsic pathways converge.
Exposure to tissue factor is not required for the activation of the intrinsic pathway. Instead, exposure of blood plasma to a negatively charged surface initiates the intrinsic cascade. There are four main components involved in the first steps of the intrinsic pathway: i) high molecular weight kininogen, a plasma protein produced by the liver ii) prekallikrein, an inactive serine protease also produced by the liver iii) factor XI iv) factor XII. All four of these molecules will associate with a negatively charged surface to initiate the intrinsic pathway. High molecular weight kininogen is found free in plasma or bound to either prekallikrein or factor VI as separate complexes. [iii][iv] Although both free prekallikrein and factor VI can bind to a negatively charged surface, the HMW kininogen complexes increases binding efficiency[v].
Upon binding to a negatively charged surface, factor XII will undergo auto-activation. Factor XIIa can now activate both prekallikrein and factor XI. Factor XII contains two cleavage sites, where cleavage of the first results in a surface-bound protease, and cleavage of the second produces a soluble protease released into the plasma[vi] Surface bound factor XIIa can activate surface bound prekallikrein and factor XI as well as plasma prekallikrein, whereas soluble factor XIIa can activate both membrane-bound and plasma prekallikrein but not factor XI[vii] Since activation of factor XI is required for continuation of the intrinsic cascade, soluble factor XIIa cannot initiate clotting. Auto-activation of factor XII is required for the intrinsic pathway to progress in vitro[viii], which might explain why soluble factor XIIa does not initiate clotting through the activation of prekallikrein in undamaged tissues in vivo. Now activated by factor XIIa, kallikrein can reciprocally activate factor XII, amplifying the activation of factor XII. Kallikrein also cleaves high molecular weight kininogen to produce bradykinin. Bradykinin acts on two different receptors, B1 and B2, the former of which is expressed only on damaged tissues. Bradykinin acts as an inflammatory mediator when stimulating B1 receptors.
Factor XIa now activates factor IX. Factor IXa assembles with calcium, factor VIIIa and factor X on an activated platelet membrane to form the intrinsic tenase complex. The intrinsic tenase complex, like the extrinsic complex, also associates with activated platelets due to the exposure of negatively charged phosphatidylserine. In this complex, factor VIIIa serves as a cofactor for the reaction and is initially activated by small amounts of either thrombin or factor Xa.
The common pathway begins with factor Xa, which forms a calcium-dependent complex with factor Va, activated by thrombin, on the membranes of activated platelets, referred to as the prothrombinase complex. The prothrombinase complex enzymatically activates prothrombin to thrombin. Thrombin then cleaves circulating fibrinogen, which is insoluble, into fibrin monomers. Formation of these monomers exposes bindings sites allowing fibrin aggregation, resulting in an insoluble fibrin meshwork. Red blood cells and platelets become trapped within this meshwork. Thrombin also activates factor XIII, and factor XIIIa crosslinks fibrin to further stabilize the clot.
Coagulation is a highly convoluted process, and genetic deficiencies of coagulation factors, drug interactions, and diseases of the bone marrow or liver may affect the clotting cascade leading to shortened or delayed clotting times. Two laboratory measurements are taken to assess the rate of blood clotting, a prothrombin time (PT) and partial thromboplastin time (PPT) test.
A prothrombin time (PT) test measures clotting initiated by the extrinsic pathway. The test is performed by centrifuging blood containing an anticoagulant that sequesters calcium (oxalate or citrate) to obtain plasma. Calcium is a co-factor required for both the extrinsic and intrinsic pathway and depleting the calcium concentration of blood will inhibit all clotting. Recombinant tissue factor, phospholipid, and an excess of calcium are then added to the plasma allowing it to clot. Tissue factor activates the extrinsic pathway, the phospholipids replace platelet phospholipid, and calcium is a cofactor required for clotting. The time it takes for the blood to clot is recorded. Because using recombinant tissue factor produced by different manufacturers results in variation of prothrombin times, each tissue factor is assigned a different International Sensitivity Index (ISI), which compares the tissue factor being used to a World Health Organization reference sample. This value is then used to generate an International Normalized Ratio (INR), which is currently used to evaluate patients. The INR is calculated by dividing a patient’s prothrombin time by a reference prothrombin time, and raising this value to the power of the ISI.
An INR above 1.4 indicates a prolonged prothrombin time, while a value below 0.8 indicates a shortened prothrombin time. A prolonged PT is diagnostic for a deficiency in or decreased production of either fibrinogen, thrombin, factor V, VII, or X. The PT test is also used clinically to monitor warfarin treatment in patients. Warfarin acts by inhibiting vitamin K epoxide reductase, which reduces vitamin K after it has been oxidized in reactions involved in the synthesis of many clotting factors. Thus, warfarin limits the reuse of vitamin K and thereby the synthesis of vitamin K dependent clotting factors.
A partial thromboplastin time (PPT) measures the rate of coagulation initiated by the intrinsic pathway. The test is performed by preparing a plasma sample as above, and then adding an excess of calcium, an activator to provide a negatively-charged surface (kaolin, eggalic acid, silica), and phospholipid. A PPT above 38 seconds indicates delayed clotting and a PTT below 27 seconds indicates a shortened clotting time. The PPT test detects deficiencies in fibrinogen, thrombin, and factors V, VIII, IX, X, XI, or XII. The PPT test is also used clinically to monitor heparin treatment in patients. Heparin is a glycosaminoglycan, a negatively charged polymer of repeating disaccharide units, and is used as an anti-coagulant. Heparin acts by binding to anti-thrombin III, which inactivates many clotting factors.
Since both PT and PPT tests were normal, there are no abnormalities in the patient’s coagulation pathways. This, however, does not rule out acute liver dysfunction. Aspartate aminotransferase (AST), a liver transaminase enzyme, was elevated. Elevated AST as well as the patient’s enlarged liver may indicate acute liver inflammation, possibly induced by heavy alcohol intake. Accessing liver function will need to be dealt with post treatment, as correcting the patients blood volume loss needs to be immediately addressed.
Hypovolemic Shock and Fluid Replacement
The cardiovascular system, consisting of the heart and blood vessels, functions to circulate blood throughout the body, allowing transport of nutrients and oxygen to and the removal of waste products from every tissue in the body. There are many homeostatic mechanisms that function to maintain appropriate blood volume and pressure to keep the organ systems adequately perfused. The blood vessels can constrict or dilate in order to raise or lower systemic blood pressure and the heart can increase or decrease both rate and strength of contraction. The kidney can also increase or decrease the filtration rate of blood. Low blood perfusion can be a result of a dysfunction in any of these homeostatic mechanisms or a decrease in blood volume to the point that they can no longer maintain proper circulation even when functioning normally. When this occurs, tissues are not receiving enough oxygen, which will eventually lead to cell death. This condition is referred to as shock, and there are three main types, each with different etiology, diagnosis, and treatments.
Cardiogenic shock is characterized by inadequate pumping of the heart without a decrease in blood volume. Failure of the heart to pump properly will decrease the circulation rate of blood, not allowing it to be distributed to the tissues quickly enough after it has been oxygen saturated in the lungs. Cardiogenic shock can be caused by any factors that significantly weaken the heart, including inflammation of the heart and overdose of drugs affecting it’s rhythm, but myocardial infarction is the most common cause. Septic shock is the result of a systemic inflammatory response to severe infection by a microbe. Localized inflammatory responses are beneficial during an infection, but when inflammatory mediators released by either our own immune cells in response to pathogens or by pathogens themselves reach high levels in systemic circulation it can have dangerous effects. Vasodilation will occur throughout the body, leading to a decrease in blood pressure and pooling of blood in the peripheral vessels. Not enough blood returns to the heart and oxygenated blood is not redistributed quickly enough. Hypovolemic shock is caused by a decrease in blood volume due to blood loss through hemorrhage, vomiting, diarrhea, or excessive urination.
Hypovolemia should be suspected in the patient because of his excessive vomiting and eventual appearance of blood in the vomit. The degree of blood loss must be assessed in order to determine the seriousness of the patients condition and to determine whether emergency treatment is necessary. The presence of black tarry stools, known as melena, should not be used to access the amount of blood loss because patients can present with melena during severe upper gastrointestinal bleeding or with as little as 100 ml of blood loss into the GI tract. Vital signs are used to approximate blood loss, most importantly blood pressure, heart rate and respiratory rate. Differential presentations of these vital signs in suspected hypovolemic patients have been used by clinicians to approximate proportion of blood volume lost. Vital signs usually do not change with 0-15% blood loss, although mild tachycardia may be observed. With 15-30% blood loss patients show marked tachycardia, tachypnea, and a decrease in pulse pressure, which is the difference between systolic and diastolic blood pressure. Systolic pressure usually does not decrease until at least 30% of blood volume is lost, and the initial drop in pulse pressure is a result of increased diastolic pressure as a result of peripheral vasoconstriction. Noticeable changes in mental state begin to occur with greater than 30% blood loss, and systolic pressure will usually be decreased by this point. If more than 40% of blood volume is lost the patient will have cold, pale skin, an almost immeasurable pulse pressure, and may become unconscious. Emergency treatment for a patient with hypovolemic shock involves ensuring proper ventilation and maximum oxygen delivery, controlling blood loss, and fluid resuscitation to replace circulating volume.
Patients airways should be assessed and they should be ventilated if it is necessary. Even if the patient is breathing regularly on their own, supplemental oxygen should be given. Once the airway has been stabilized fluid resuscitation should be administered. Before describing the different types of resuscitation fluids and how to administer them, it is important to understand the physiology of fluid distribution between body compartments first.
Body Fluid Physiology
About 60% of a person’s total body weight is contributed to by water, but this proportion decreases with age and is lower in women and obese individuals due to their higher fat content. At an average weight of one kilogram per liter, there are about 0.6 liters of body fluid per kilogram of body weight. This fluid is distributed into two main compartments. The first compartment consists of all the fluid that is located extracellularly, and contains 45% of the body’s water at homeostatic conditions. The second compartment is intracellular fluid, and contains the body’s remaining 55% of water. Although the intracellular space is not a true compartment, as it consists of trillions of individual cells, it is treated as one due to the similarity of the intracellular environment, plasma membrane, and response to changes in the extracellular fluid of all the cells in the body. When exogenous fluids are administered directly into the bloodstream they will distribute throughout these compartments and alter existing fluid concentrations based on their osmolarity and tonicity.
Osmolarity is a measure of the solute concentration of a liquid, and is determined by the concentration of all solutes regardless of their molecular weight. When liquids of differing osmolarity are mixed, solutes will diffuse throughout the new volume until osmolarity has equilibrated. However, if solutions of differing osmolarity are separated by a semi-permeable membrane, not all solutes can diffuse across the membrane and tonicity becomes the major equilibrating factor. Tonicity is most often described as the effective osmotic force, and is dependent only on the concentration of solutes that are impermeable to the membrane a liquid is surrounded by. All permeable solutes and water (if it is permeable) will diffuse across the membrane in order to equilibrate osmolarity. The concentration gradient of impermeable solutes across the membrane determines the diffusion of water across it. Understanding the difference between these two terms is important in physiology because you can have resuscitation fluids with the same osmolarity as but different tonicity than extracellular fluid.
Extracellular fluid can be divided into many smaller compartments, each with unequal distributions of total body water (TBW). Plasma (7.5% TBW) makes up the intravascular portion of extracellular fluid, and its fluid distribution will become extremely important when discussing fluid resuscitation. Water retained by the red and white blood cells is considered part of the intracellular fluid compartment because of their plasma membranes. Interstitial fluid (20% TBW) is located between tissues and blood vessels, which is known as the interstitial space. All non-endothelial cells of the organs are covered by interstitial fluid. Dense connective tissue (7.5% TBW) and bone (7.5%) both contain significant amounts of water in their interstitial spaces, but the fluid dynamics for both of these compartments are extremely slow so their volumes become irrelevant when dealing with immediate fluid resuscitation. Transcellular fluid (2.5% TBW) is contained in a few epithelial-lined compartments of the body and includes cerebrospinal fluid, synovial fluid, aqueous humor, and vitreous humor. Transcellular fluid composition and volume is mostly determined by the active transport of epithelial cells, and the occluding junctions of the epithelium serves to keep diffusion of fluids into transcelluar compartments at a minimum.
Since bone, dense connective tissue, and transcellular fluid do not equilibrate rapidly they are not important when considering immediate fluid replacement. This makes interstitial fluid and plasma the compartments to be interested in when considering extracellular fluid during fluid resuscitation. Distribution of fluid between these two compartments is dependent on their relative volumes and the semi-permeable membrane between them, which is the endothelial lining of the capillaries. Capillaries are permeable to most solutes in order to allow the diffusion of important nutrients, hormones, and gases to and from the tissues. It is important that excess fluid does not buildup around the tissues though, and there is a physiological mechanism that ensures this.
Two factors, the hydrostatic and osmotic pressure, determine whether fluid will move into or out of capillaries at their arteriolar and venular ends. Hydrostatic pressure is the force a fluid exerts on the walls of it’s container, in this case the endothelium, and is directly proportional to volume. Hydrostatic pressure is always higher inside capillaries so the net hydrostatic force pushes fluid out. The opposing osmotic force is produced by circulating plasma proteins, mostly albumin, that do not easily cross through capillaries and produce an osmotic gradient between the plasma and interstitial fluid. The osmotic force exerted by these proteins, which is referred to as oncotic pressure, serves to pull water back into capillaries. At the arteriolar end of capillaries the hydrostatic pressure is always larger than oncotic pressure, which results in net movement of fluid into the interstitial fluid. As fluid leaves the capillary the volume decreases and consequently lowers hydrostatic pressure. At the venular end of capillaries the oncotic pressure is now larger than the reduced hydrostatic pressure, resulting in a net movement of fluid into the capillaries.
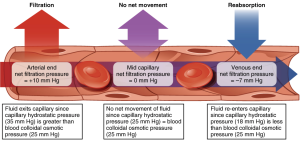
Note that colloid osmotic pressure (oncotic pressure) remains constant while only hydrostatic pressure decreases.
The intracellular compartment is separated from the interstitial fluid by semi-permeable cell membranes. Small nonpolar molecules are able to diffuse directly through plasma membranes, but large and charged molecules require either active transport or membrane protein channels in order to cross the membrane. This selective permeability determines the distribution of fluid into cells from the extracellular fluid. Any volume of fluid that is completely permeable to cell membranes will distribute into cells based on it’s relative volume to extracellular fluid. Volumes with differing tonicity, however, can remain extraceullarly or draw water into or out of cell.
There is also a third compartment that does not normally hold a significant proportion of body water, but fluid may accumulate here in certain diseased states. This compartment, known as the third space, includes all body cavities such as the peritoneal or pleural cavity. These compartments are lined by serous membranes, composed of epithelial cells, and the small amount of fluid naturally present in them usually acts simply as lubricant. Any accumulation of excess fluid in the third space is non-functional, and is usually the result of excessive tissue edema.
Resuscitation Fluids
In deciding which fluid preparation to use during resuscitation there are two main types of intravenous fluids to choose from, either a crystalloid or colloid solution. Crystalloid solutions contain ions with or without glucose, whereas colloid solutions contain proteins or high molecular weight polymers that maintain the oncotic pressure of blood once in circulation. The tonicity and oncotic pressure of these fluids will determine their distribution throughout the body compartments. Colloid solutions remain intravascularly because they maintain the oncotic pressure of blood, creating no osmotic gradient across the endothelium. Crystalloid solutions will quickly equilibrate with the interstitial fluid and, depending on the tonicity, the intracellular compartment. Because of this, a larger volume of crystalloid is required to maintain blood volume when compared to colloid solutions. Physicians may also mix crystalloid and colloid solutions depending on the circumstance. There are many benefits and disadvantages to both crystalloids and colloids, and there has been much debate over which is more effective in fluid resuscitation. Colloids are more efficient at replacement of lost blood volume but are much more expensive than crystalloid solutions. I am going to go through some examples of administering different types of fluids intravenously to demonstrate their distribution.
Colloids have the simplest distribution, because they maintain the same oncotic pressure as blood they remain intravasularly. If the solution is isotonic, then no fluid will enter the red or white blood cells and the entire volume administered will remain in plasma. An isotonic crystalloid solution will easily pass through capillary walls, but because it has the same tonicity as cells there is no osmotic force that will drive it intracellularly. This will lead to a distribution of the fluid between the interstitial fluid and plasma based on their relative volumes, which is about 3:1. This will result in only 25% of the administered fluid remaining intravascularly, and is why you must administer three to four times the volume of blood lost in order to replace it when using crystalloids. If glucose is present in a solution it will be rapidly taken up by cells and have no effect on the solution’s tonicity. Since glucose does not effect tonicity, a pure glucose solution would distribute between the intracellular and extracellular compartments based on their proportional volumes, which are about 2:1. This will result in 66% of the administered fluid being taken into cells and 33% distributed between the interstitial fluid and plasma. This leaves about 25% of the fluid in the interstitium and 8% in the plasma. Because fluid preparations distribute differently throughout the body based on their composition, choosing a solution most appropriate to a patients needs is extremely important.
In a hypovolemic patient, either isotonic saline or Ringer’s lactate solution are the fluid of choice to begin resuscitation. Ringer’s lactate is an isotonic solution consisting of salts (Na+, K+, Cl– & Ca2+) and lactate. No distinct advantage has been shown in using crystalloids versus colloids in shock patients, but a higher mortality rate has been found in critically ill patients when albumin has been used for resuscitation. [ix] The increased risk of albumin solutions and greater cost of colloids may be why more physicians prefer using crystalloids in shock patients. In pig models, using isotonic saline to treat hemorrhagic shock results in hyperchloremic acidosis; a smaller amount of Ringer’s lactate is also required to stabilize blood pressure than saline. [x] This demonstrates advantages to using Ringer’s lactate over isotonic saline during resuscitation. In hemorrhagic shock, hypoperfusion of the organs will lead to an increase in anaerobic metabolism and a buildup of lactate in the blood. This buildup commonly causes metabolic acidosis in shock patients. If isotonic saline is administered, the induced hyperchloremia can exacerbate existing acidosis. Ringer’s lactate avoids this complication. Substituting pyruvate for lactate in Ringer’s solution can correct metabolic acidosis during shock in animal models. [xi] A new pyruvate Ringer’s solution may be ideal in fluid resuscitation of shock patients.
Blood Typing
While initial fluid replacement is occurring, patient blood type should be determined in order to perform a transfusion. Blood types are grouped based on the different alleles of antigenic determinants expressed on the surface of red blood cells, or erythrocytes. There are over 300 antigens expressed on erythrocyte membranes, but only a few are important in blood transfusions. The ABO and Rhesus antigen systems are the ones considered during blood typing because they are the most immunogenic, meaning they illicit stronger immune responses, which want to be avoided.
The ABO system consists of two carbohydrate antigens, A and B, and individuals can express one of them, both of them, or neither on their erythrocyte membranes. These genotypes result in the blood groups A, B, AB and O (neither expressed). During development of the immune system, only lymphocytes with specificity to foreign antigens will reach maturity. This is because every antigen specific immune cell is exposed to self-antigens during development, and if they react strongly to self-antigens they are stimulated to undergo apoptosis. Therefore, individuals that do not express the A or B antigen will develop antibody-producing cells, or B cells, specific for them. Antibodies against the A or B antigens develop early in life, and this is thought to be a result of exposure of B cells to analogous antigens located on bacteria or in food. This means that there are already antibodies in the plasma of children and adults that will illicit an immediate immune response without the delay that follows initial exposure to antigen. It is important that antibodies, either from the recipient or donor, not be mixed with erythrocytes expressing corresponding antigens. If this happens, the antibodies will bind to their antigens and lead to agglutination and hemolysis of erythrocytes. Blood typing is performed in order to avoid these hemolytic reactions so that transfused blood has a maximal effect of restoring lost erythrocytes. If a small amount of blood is needed, transfusion of whole blood is appropriate to use in order to restore lost erythrocytes even if hemolytic reactions will occur. If a large volume is required, however, isolated packed red blood cells are used to restore erythrocytes and avoid antibody antigen interactions.
The Rhesus antigen group is the second to be considered in blood typing, and consists of 46 antigens. Only the expression of the D Rhesus antigen, however, is important for blood typing. Individuals with the D antigen are Rh+, and those without it are Rh-. Antibodies will develop in Rh- individuals against the D antigen. Development of these antibodies first requires exposure to the D antigen, which does not occur naturally.
There are disadvantages to using packed red blood cells for transfusion. Many changes occur during storage of blood which can effect oxygen offloading in the tissues. The maximum storage time for blood is 42 days, but it is recommended that it be administered 14 days or less after storage. Lack of ATP in stored blood will cause stiffening of erythrocyte membranes. [xii] Erythrocytes have about the same diameter as that of capillary walls, but are very flexible in order to squeeze through them to deliver oxygen. A more rigid membrane makes it more difficult to pass through capillaries and diminishes oxygen delivery. There are also lower levels of 2,3-bisphosphoglycerate (2,3-BPG) in stored red blood cells. 2,3-BPG decreases the affinity of hemoglobin for oxygen, and contributes to oxygen offloading in the tissues. Stored erythrocytes with lower 2,3-BPG levels will have higher affinity for oxygen in the tissues and this will decrease offloading. In order to avoid these disadvantages, many hemoglobin based oxygen carriers have been put into development, although none are in current clinical use. These oxygen carriers consist of either a solution containing isolated or recombinant hemoglobin. The appeal of these resuscitation fluids is their non-antigenicity, high oxygen carrying capacity, and longer shelf life.
Post Resuscitative Treatment
Once correction of shock has begun using resuscitation fluids in a patient with suspected upper gastrointestinal bleeding, steps should be taken in order to stop ongoing hemorrhage, identify the bleeding site, and reduce the risk of re-bleeding. Administering a nasogastric lavage removes blood lost into the stomach and allows clinicians to monitor the extent of ongoing blood loss. Proton pump inhibitors reduce gastric acid secretion and significantly decrease the chance of re-bleeding. Somatostatin, or it’s synthetic analog octreotide, reduce bleeding through their vasoconstrictive effects. The bleeding site can then be definitively identified and treated directly by endoscopy. In patients who do not respond to the above treatments, surgical intervention may be necessary.
Insertion of a nasogastric tube can be performed immediately after infusion of resuscitation fluids has begun. Confirmation that the tube is in the stomach can be obtained by air insufflation, where a small amount of air is injected into the tube with a suction syringe and a bubbling sound is listened for in the upper left quadrant. Once the tube is in place, the stomach is irrigated by injecting small amounts of saline into the tube and then suctioning fluid out of the stomach. Aspirate from the stomach can have a blood red or coffee ground appearance, and may even remain clear if the site of bleeding is duodenal or bleeding has stopped. Stomach aspirate as well as stool color are good indicators of mortality rate in upper gastrointestinal bleeding patients. [xiii] The volume of aspirate removed also gives physicians a further estimate of blood loss and can be used to decide whether a blood transfusion should be performed. A retrospective study found no difference in mortality rate or risk of re-bleeding in gastric hemorrhage patients not administered a nasogastric lavage versus those who were, but those who did were associated with earlier time to endoscopy. [xiv] Whether the use of a nasogastric lavage is necessary in gastric hemorrhage patients should be considered by physicians, as it does not decrease mortality and causes a great deal of patient discomfort.
Intravenous infusion of proton pump inhibitors (PPIs) may also be administered to a patient as soon as fluid resuscitation has begun. PPIs maintain a stomach pH of 6 by directly blocking secretion of protons into the gastric lumen by parietal cells. The increased pH does not do anything to stop ongoing hemorrhage, but significantly reduces the risk of a re-bleeding incident. Because of this effect, infusion of PPIs is often continued in patients after the bleeding site has been treated.
Both somatostatin and its analog octreotide are used to reduce gastric bleeding. Somatostatin is a hypothalamic hormone with inhibitory functions at many target tissues. Release of the pituitary hormones somatotropin and thyrotropin are suppressed by the action of somatotstatin. Somatotstatin is also released by the delta cells of pancreatic islets in response to low pH during digestion and inhibits the release of many gastrointestinal hormones. The GI hormones involved with somatostatin’s reduced bleeding rate effect are insulin and glucagon.
Somatostatin is initially synthesized as a preprohormone, which is proteolytically cleaved within the secreting cell to prosomatostatin. Prosomatostain contains two cleavage sites, and cleavage at either site results in a biologically active peptide. Both active forms act on all five somatostatin receptors, but the 28 amino acid form (SS-28) has a more prolonged suppression effect than the 14 amino acid form (SS-14). [xv]
Somatostatin’s vasoconstrictive effects on the splanchnic vasculature are likely mediated indirectly as there are no somatostatin receptors on endothelial or vascular smooth muscle cells. Inhibition of insulin and glucagon may be somatostatin’s most potent effect on the splanchnic vasculature as both of these hormones increase splanchnic blood flow. [xvi] Suppression of these pancreatic hormones may lessen their effect on splanchnic blood flow resulting in decreased perfusion to the splanchnic organs. Octreotide is an octapeptide analog of somatostatin that is currently used in place of somatostatin for treatment of gastric bleeding. Octreotide acts on all somatostatin receptors and is a more potent inhibitor of insulin, glucagon, and growth hormone. The inhibitory effects of somatostatin and octreotide on other gastrointestinal hormones, such as secretin and gastrin, reduce acid secretion into the stomach and may contribute to a reduced risk of re-bleeding.
Endoscopy is a procedure used to both identify the gastrointestinal bleeding site as well as treat it. An endoscope is a flexible tube that allows visualization of the gastrointestinal tract through either a lens and eyepiece system or a camera. The endoscope is used to identify the bleeding site in a patient and can be used to treat it directly. There are many endoscopic therapies that can be used in order to treat gastric bleeding. If the cause of bleeding is due to ruptured varices, which are unusually dilated blood vessels, band ligation is the most common treatment. Varices of the gastrointestinal vasculature are usually a result of portal hypertension, where blood pressure in the portal vein leading from the splanchnic organs to the liver increases and leads to backflow into the venous system. Band ligation is performed by fastening a device to the tip of an endoscope that holds an elastic band. The band is placed over hemorrhaging varix and constricts around it. This occludes blood flow to the hemorrhage site on the vessel, and this tissue eventually undergoes necrosis and is sloughed into the gastric acid.
Many other instruments can also be fastened to the end of an endoscope. Doctors can equip the scope with an injection needle, through which different pharmaceuticals can be used to treat gastric bleeding. Sclerosing agents are often injected, which cause inflammation and subsequent fibrosis of veins, occluding their lumen and blocking blood flow through them. Other instruments are used to perform coagulation therapies. Argon plasma coagulation, for an example, funnels argon gas down the endoscope tube where it is ionized by a discharge device on the tip of the endoscope. This causes an arc adjacent to the hemorrhaging tissue and stimulates coagulation. Most other coagulation therapies employ a technique to raise the temperature of the bleeding site in order to stimulate coagulation, which will occur starting at 60° C.
Once the patient’s hypovolemia has been corrected and bleeding has been stopped, emergency treatment is over. Monitoring of cardiac and kidney function should continue and the patient should be kept over night for observation. It is important to speak with the patient about his drinking habits and inform him that if he continues with this behavior it could result in a re-bleeding incident. The patient should be referred to a psychological counselor to help him through his divorce with more appropriate behavior.